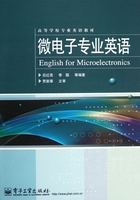
6.1 Recombination
The thermodynamic nonequilibrium excess chargescan be present in the semiconductor. They can be created by carrier injectionthrough contacts,an electron beam or the absorption of light with wavelength smaller than the bandgap. After the external excitation is turned off,the semiconductor will return to the equilibrium state. The relaxation of carriers into energetically lower states(and energy release)is called recombination . The term stems from the electron recombining with the hole created after absorption of a photon.
However,there are other recombination mechanisms.
6.1.1 Band to Band Recombination
The band-band recombinationis the relaxation from an electron in the conduction band into the valence band(the empty state there is the hole). In a direct semiconductor,electrons can make an optical transition between the bottom of the conduction band to the top of the valence band. In an indirect semiconductor,this process is only possible with the assistance of a phonon and is thus much less probable.

Fig.6.1 Processes of band band recombination:(a)spontaneous emission,(b)absorption and(c)stimulated emission. A full(empty)circle represents an occupied(unoccupied)electron state.
Fig.6.1(a)shows the processes of the spontaneous recombinationof an electron of energy Eeand a hole of energy Eh. A similar consideration is made for the absorptionprocess [Fig.6.1(b)]. An electron is transferred upon light absorption from a valence-band state(occupied)to a conduction-band state that must be empty. The process is proportional to the light intensity.
In stimulated emission,an incoming photon triggers’ the transition of an electron in the conduction band into an empty state in the valence band. The emitted photon is in phase with the initial photon [Fig.6.1(c)].
6.1.2 Free-Exciton Recombination
The observation of free-excitons is limited for semiconductors with a small exciton binding energies(such as in GaAs)to low temperatures. However,for large exciton binding energy,recombination from free-excitons is observed even at room temperature,as shown in Fig.6.2 for ZnO.
6.1.3 Auger Recombination
In competition with the radiative,bimolecular recombination is the Auger recombination(Fig.6.3). In the Auger process,the energy that is released during the recombination of an electron and hole is not emitted with a photon but,instead,transferred to a third particle. This can be an electron [eeh,Fig.6.3(a)] or a hole [hhe,Fig.6.3(b)]. The energy is eventually transferred nonradiatively from the hot third carrier via phonon emission to the lattice.
The probability for such process is ∝ n 2p if two electrons are involved and ∝ np 2 if two holes are involved. The Auger process is a three-particle process and becomes likely for high carrier density,either through doping,in the presence of many excess carriers,or in semiconductors with small bandgap.
Auger recombination is the inverse of the impact ionization .

Fig.6.2 Charge-carrier distribution during inversion,necessary for lasing. Shadedareas are populated with electrons. A stimulated transition between an electron and a hole is indicated.

Fig.6.3 Schematic representation of Auger recombination. An electron recombines with a hole and transfers the energy to(a)another electron in the conduction band,(b)another electron in the valence band.
6.1.4 Band-Impurity Recombination
Another recombination process is the capture of carriers by impurities. This process is in competition with all other recombination processes,e. g. the radiative recombination and the Auger mechanism. The band-impurity recombinationis the inverse process to the carrier release from impurities. It is particularly important at low carrier densities,for high dopant concentration and in indirect semiconductors since for these the bimolecular recombination is slow. The theory of capture on and recombination involving impurities is called Shockley-Read-Hall(SRH)kinetics.
An example of-band impurity recombination is shown in Fig.6.4
6.1.5 Surface Recombination
A surface is typically a source of recombination,e. g. by midgap levels induced by the break of crystal symmetry. The surface recombinationvelocity for GaAs is shown in Fig.6.5. For InP,if the surface Fermi level is pinned close to midgap,the surface recombination velocity increases from ~ 5 × 103 cm/s for a doping level of n ~ 3×1015 cm-3 to ~ 106 cm/s for a doping level of n ~ 3×1018 cm-3 . For Si,the surface recombination rate depends on the treatment of the surface and lies in the range between 10~104 cm/s . The Si-SiO2 interface can exhibit S ≤ 0.5 cm/s.

Fig.6.4 Band-to-impurity processes at an impurity with one level(left:initial,right:final state in each part):(a)electron capture(from conduction band),(b)electron emission(into conduction band),(c)hole capture(from valence band),(d)hole emission(into valence band). The arrows indicate the transition of the electron.

Fig.6.5 Surface recombination velocity for GaAs as a function of n-type doping concentration. Different experimental points correspond to different surface tre atment methods. Dashed line is a guide to the eye.