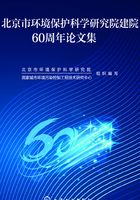
Historical and future emission of hazardous air pollutants(HAPs)from gas-fired combustion in Beijing,China
Yifeng Xue1,2,Lei Nie1,Zhen Zhou1,Hezhong Tian2,3,Jing Yan1,Xiaoqing Wu1,Linglong Cheng1
1. National Engineering Research Center of Urban Environmental Pollution Control,Beijing Municipal Research Institute of Environmental Protection,Beijing 100037,China.
2. State Key Joint Laboratory of Environmental Simulation & Pollution Control,School of Environment,Beijing Normal University,Beijing 100875,China.
3. Center for Atmospheric Environmental Studies,Beijing Normal University,Beijing 100875,China.
Abstract:The consumption of natural gas in Beijing has increased in the past decade due to energy structure adjustments and air pollution abatements.In this study,an integrated emission inventory of hazardous air pollutants(HAPs)emitted from gas-fired combustion in Beijing was developed for the period from 2000~2014 using a technology-based approach.Future emission trends were projected through 2030 based on current energy-related and emission control policies.We found that emissions of primary HAPs exhibited an increasing trend with the rapid increase in natural gas consumption.Our estimates indicated that the total emissions of NOX,PM10,PM2.5,CO,VOCs,SO2,BC,Pb,Cd,Hg,As,Cr,Cu,Ni,Zn,PCDD/Fs,and B[a]P from gas-fired combustion in Beijing were approximately 22422 t,1042 t,781 t,19097 t,653 t,82 t,19 t,0.6kg,0.1kg,43kg,52kg,0.3kg,0.03kg,4.3kg,0.6kg,216μg,and 242 g,respectively,in 2014.To mitigate the associated air pollution and health risks caused by gas-fired combustion,stricter emission standards must be established.Additionally,combustion optimization and flue gas purification system could be used for lowering NOX emissions from gas-fired combustion,and gas-fired facilities should be continuously monitored based on emission limits.
Key words:gas-fired combustion;hazardous air pollutants(HAPs);emission inventory;temporal trends;spatial variation
1 Introduction
Beijing,the capital of China,lives with an enormous population(over 21 million)and has undergone rapid economic growth,resulting in considerable energy consumption and serious environmental problems(Huang et al.,2014;Sun et al.,2016).In recent years,Beijing has adopted numerous measures to control and improve air quality,such as shutting down coal-fired power plants,exchanging coal-fired industrial boilers for clean energy options,and encouraging residential cooking using natural gas(He et al.,2014;Lin et al.,2016;Liu et al.,2016b;Xue et al.,2016).Such measures have led to obvious variations in the energy consumption structure of Beijing.As a result,coal use has decreased annually,while both the volume and proportion of natural gas consumption have increased rapidly.
Natural gas is regarded as a relatively clean energy compared to coal-fired combustion,as gas-fired combustion emits fewer air pollutants under uncontrolled conditions.However,coal-fired combustion with the use of high-efficiency gas purification systems can reach lower emission,even less than gas-fired combustion which released directly into the atmosphere.NOX emissions released by gas-fired combustion cannot be ignored.Compared to coal-fired combustion studies(Hu et al.,2013;Liu et al.,2016;Pei et al.,2016;Tian et al.,2010;Wang et al.,2010;Xue et al.,2016),few studies have focused on the temporal trends and spatial variations in emissions from gas-fired combustion(Liu et al.,2016,Makonin et al.,2016;McJeon et al.,2014).Accurately estimating the emission of various HAPs from gas-fired combustion is essential for improving air quality management and policy.
The purpose of this study is to improve upon the existing knowledge described above by developing a unit-based emission inventory for gas-fired combustion using a bottom-up methodology.We analyzed the historical trends in gas-fired combustion in Beijing from 2000 to 2014 and calculated the typical HAPs emissions using detailed source-specific information from gas-fired boilers,including boiler type,flue gas purification technology,etc.Furthermore,we projected future emissions from gas-fired combustion through 2030 using a scenario analysis and proposed a strategy for reducing emissions to provide a reference and basis for environmental management and decision making.
2 Material and methods
2.1 Study domain
Beijing,the capital of the People’s Republic of China,is geographically located at 39°56'N and 116°20'E on the northwestern edge of the North China Plain.It is surrounded by the Taihang and Yanshan Mountains to the west,north,and northeast(Gao et al.,2014).Beijing encompasses a total area of 16,410.54km2 and is divided into 16 administrative districts with approximately 21,148,000 residents.With the improvement of living conditions and standards,the demands for energy in the power,industrial production,and residential heating and cooking sectors has increased(Liu et al.,2016;Zhang et al.,2016),which has threatened energy security and caused serious environmental pollution.
2.2 Emission inventory calculation
Gas-fired combustion was divided into three types:gas-fired power boilers,gas-fired industrial boilers for industrial production and heating,and gas-fired stoves for residential cooking and heating.Annual emissions of various HAPs from gas-fired power and industrial boilers were calculated using boiler-specific data on natural gas consumption and emission factors(EFs)associated with the applied control technologies,which were determined based on field measurements and investigation.The algorithm for the bottom-up emission inventory of primary air pollutants associated with gas-fired combustion can be expressed using the following equation:
where the subscripts i and j represent the HAPs type and combustion type,respectively;CEF is the comprehensive EF of each pollutant(including PM10,PM2.5,SO2,NOX,VOCs,BC,heavy metals,PCDD/Fs and B[a]P);and represents the average removal efficiency of control technologies for various pollutants.
2.2.1 Activity data
Energy use data,including data on historical coal and natural gas consumption,were obtained from the Beijing Statistical Yearbook.Consumption information and the types of gas-fired power boilers and industrial boilers were obtained by conducting field investigations organized by the Beijing Environmental Protection Bureau(BJEPB).These records were then cross-referenced with a comprehensive analysis of annual statistics(BMBS,2014).A database of gas-fired boilers was compiled at the unit level.We collected detailed point source information from 2572 gas-fired industrial enterprises and eight gas-fired power plants.The detailed information included the latitude and longitude of each boiler,boiler type,installed capacity,starting year of operation,annual consumption of natural gas by each unit,installed emission control technologies,and starting time of each technology.The geographical locations of the gas-fired industrial boilers and power plants distinguished by starting year are illustrated in Fig.1.

Fig.1 Geographical locations of gas-fired industrial enterprises and power plants in Beijing
As shown in Fig.2,coal and natural gas consumption varied considerably between 2000 and 2014.The figure reveals that coal consumption has decreased in the power,industrial,and residential sectors,mainly due to the management policies and coal pollution controls in the past decade.These controls include the gradual shutdown of coal-fired power plants,bans on building new coal combustion devices,and elimination of coal-fired boilers for industrial production.Meanwhile,with the replacement of coal combustion,the consumption of natural gas has increased at an annual rate of 18.2%,which is higher than the rate of decline in coal combustion.The continuously increasing natural gas supply is not only being used for industrial boilers and power boilers to generate community heat and electricity,but also used in residential cooking and heating instead of coal.

Fig.2 Temporal variations in coal and natural gas consumption in Beijing from 2000~2014
2.2.2 Emission factors
NOX emissions are one of the main concerns associated with gas-fired combustion.NOX EFs are significantly influenced by unit capacity,boiler type,combustion operation conditions,and control measures.The local EF of uncontrolled NOX from industrial boilers,which was calculated according to field measurements of flue gas on the stack,was observed in a previous study(Xue et al.,2014).Furthermore,we collected all the hourly controlled NOX emission concentrations and flue gas volumes from the continuous emission monitoring systems(CEMS)of gas-fired power units which was installed on the stack.These values were then combined with information regarding natural gas consumption to calculate the EF of NOX from gas-fired power boilers(see Fig.3).We adopt NOX EFs for gas-fired residential stoves recommended by the State Environmental Protection Administration(SEPA)of China.These values are generally regarded to represent realistic emission levels for gas-fired residential stoves in Beijing(SEPA,2008).

Fig.3 EF of NOX from gas-fired power boilers
The EF of particulate matter(PM)is dependent on the characteristics of unabated emissions from the overall combustion process.Black carbon(BC)originates from fossil fuels,and the properties of the resulting BC,such as atmospheric lifetime and optical properties,are dependent on the fuel properties and combustion conditions.Due to the lack of actual measurement data,we adopted field measurements of PM10,PM2.5,SO2,VOCs and BC for uncontrolled gas-fired boilers and residential stoves from the US EPA(US EPA,1998)in this study.Most of heavy metals(HMs)considered are normally released as compounds(e.g.,oxides and chlorides)in association with particulates that originate from the contents and purities of natural gas.The EFs of other HAPs associated with gas-fired facilities,such as Pb,Cd,Hg,As,Cr,Cu,Ni,Zn,PCDD/Fs,and B[a]P,are referenced from the EEA(EEA,2013).The average EFs of various hazardous air pollutants are summarized in Table 1.
Table 1 Emission factors of various air pollutants from gas-fired combustion

Notably,the yearly EFs of NOX,PM,SO2,VOCs and HMs from 2000 to 2014(see Fig.4)changed with technological innovation and development.This trend can be regarded as a uniform model based on the variation in the actual concentration of NOX from gas-fired boilers between 2000 and 2014.Because there were no significant variations in the emission controls of other HAPs from 2000 to 2014 and the results of local field tests are not available to determine yearly variations in EFs,we use constant average EFs to represent the overall emission scenario during the period.This introduces some uncertainties into our emission inventories,which are discussed in the uncertainty analysis section.

Fig.4 Temporal variations in EFs associated with gas-fired combustion in Beijing
2.3 Scenario analysis
Future pollution trends are projected using three emission scenarios:one business-as-usual(BAU)scenario and two improved pollution control(IPC-1 and IPC-2)scenarios.The consumption of natural gas would depend on the economic development,the requirement by environmental policies and energy supply and security.Based on difference scenarios,we forecasted the future consumption trend of natural gas consumption.In the BAU scenario,we assume that natural gas consumption in Beijing will continue to be associated with industrial boilers in accordance with existing energy policies.All enforcement efforts are treated the same as those in previous years.Additionally,all current regulations,end-of-pipe control strategies,and implementation efforts would continue from 2013 to 2030.In the IPC-1 scenario,we assume that new energy policies are introduced and enforced.This scenario would result in structural energy adjustments,energy efficiency improvements,enhanced enforcement of environmental standards,widespread and effective use of flue gas cleaning systems,and mitigation of the final emission levels of various air pollutants below the local standards in Beijing.In the IPC-2 scenario,we assume that newly revised emission standards for air pollutants from industrial boilers will be issued that are comparable to those of the EU or US EPA and that the best available control technology(BACT)will be installed in industrial gas-fired boilers.These measures would further lower the final emission levels of various air pollutants and reduce coal consumption in industrial boilers by replacing coal with natural gas and electricity to meet energy demands and improve air quality.
3 Results and discussion
3.1 Historical emission inventory from gas-fired combustion
Emissions of primary air pollutants from gas-fired combustion were calculated via the EF approach described above,as summarized in Fig.5.The annual emissions from gas-fired combustion varied with natural gas consumption,exhibiting gradual increasing trends in each year,except in 2011,which was due to a shortage in the natural gas supply and the slowdown in economic growth of Beijing in 2011 compared with last year,the intensity of industrial production activity has decreased,for example power generation decreased by 2.2%.In addition,energy efficiency have been improved,energy consumption per unit gross domestic product(GDP)has also declined from 58 tons standard coal/million Yuan RMB in 2010 to 0.46 in 2011.Under the combined effect of these factors,the consumption of natural gas in 2011 has been reduced,and the emissions of HAPs from gas-fired combustion have also been reduced.

Fig.5 Historical HAPs emissions from gas-fired combustion in Beijing from 2000~2014
In the power sector,only two gas-fired plants and three generators were installed in 2006.However,this sector reached eight gas-fired plants and twenty generators installed by the end of the period,causing the consumption of natural gas to increase from 31.4 million cubic meters in 2006 to 357.5 million cubic meters in 2014,representing an annual average growth rate as high as 35.5%.One year before holding the 2008 Olympics,new local standards were issued in Beijing for emissions from boilers(DB11/139—2007)to improve the air quality,and new natural gas-fired power plants were required to install low-NOX burners.Subsequently,an increasing number of gas-fired power plants gradually launched denitration retrofits,including SCR and other techniques,to satisfy the local emission standards.Consequently,the NOX emission level from gas-fired power plants dropped,and the yearly growth rate of NOX emissions was 23.6% lower than the growth rate of natural gas consumption.However,the emissions of other air pollutants increased at similar growth rates because no obvious changes in emission controls occurred.
Driven by clean energy alternatives to industrial coal-fired boilers,the number of natural gas-fired boilers and the associated installed capacity exhibited high growth rates.Before 2008,these growth rates were relatively slow.However,the consumption of natural gas rapidly increased in 2008,2012,and 2014 due to the implementation of temporary control measures for the Olympic Games and the Clean Air Plan.During these periods,reducing HAPs emissions from coal combustion for industrial and community heating was the major control target to combat the serious air pollution in Beijing.Fewer control measures focused on gas-fired industrial boilers because natural gas combustion was considered as a relatively clean energy.Therefore,the yearly growth rate of air pollutant emissions was approximately 14%,mimicking the increase in the consumption of natural gas.On the other hand,natural gas consumption by residential gas facilities remained stable with the development of the natural gas network.Due to lack of emission standards for residential stoves and wall-mounted heaters,the associated levels of direct emissions have minimally changed,maintaining a 13.5% growth rate in annual emissions from residential gas combustion between 2000 and 2014.
The estimates indicated that the emissions of NOX,PM10,PM2.5,CO,VOCs,SO2,BC,Pb,Cd,Hg,As,Cr,Cu,Ni,Zn,PCDD/Fs,and B[a]P from gas-fired combustion in 2014 were approximately 22422 t,1042 t,781 t,19097 t,653 t,82 t,19 t,0.6kg,0.1kg,43kg,52kg,0.3kg,0.03kg,4.3kg,0.6kg,216μg,and 242 g,respectively.According to calculations by the Beijing Municipal Research Institute of Environmental Protection(BMRIEP,2016),NOX emitted from natural gas combustion accounted for approximately 13% of overall NOX emissions in Beijing.Particularly,NOX from natural gas combustion accounted for 23.4% of total NOX emissions in the heating season and 6.2% in the non-heating season.Thus,the combustion of natural gas contributes to a certain proportion of the total NOX emissions,especially in winter,and a focus should be placed on reducing pollutant emissions associated with natural gas combustion via various control measures.
3.2 Temporal and spatial distributions of gas-fired emissions in Beijing
3.2.1 Spatial distribution
The spatial distributions of emissions from gas-fired boilers were based on boiler unit locations,and they were determined for 1km×1km grids in Beijing.Residential gas combustion was allocated based on the population distribution.Fig.6 shows the gridded geographical distribution of NOX emissions from gas-fired combustion(including emission from the power sector,industrial sector,and residential sector)at a 1km×1km resolution.

Fig.6 Spatial distribution of NOX emissions from gas-fired combustion in Beijing in 2014
Emissions of primary air pollutants from gas-fired power boilers in Beijing are predominantly concentrated in central urban areas.These areas require electricity and heat for large populations,and local generation prevents electricity loss due to long-distance transfers.The spatial distribution of gas-fired industrial boilers is closely related to the economic development levels,populations,energy supplies,industrial production levels,and winter concentrated heating demands in the 16 different prefecture districts.Similarly,residential gas combustion was concentrated near sites of intensive human activities in urban areas.
According to the spatial distribution of the total emissions from gas-fired combustion,Chaoyang district contributed to the highest percentage of NOX emissions(21.9%)due to the large number of gas-fired generators located in the district.As an urban expansion area,Haidian district contributed to the second highest percentage of NOX emissions(18.9%)due to the large number of gas-fired industrial boilers and the large population in the district.Xicheng district is an urban downtown center with a dense population and numerous commercial enterprises that require considerable power and heating.However,there are no coal-fired industrial boilers in this urban downtown district due to a policy banning coal combustion.Nevertheless,the consumption of natural gas was relatively high,and the NOX emissions from gas-fired combustion totaled approximately 2819t,accounting for 12.6% of overall natural gas combustion emissions in Beijing.Correspondingly,rural areas such as Men Tougou district,Miyun district,and Yanqing district are largely affected by geographic conditions that hinder the installation of natural gas pipes,resulting in low natural gas consumption and low NOX emissions associated with natural gas combustion.Thus,the emissions from coal combustion in these surrounding districts are much higher to fulfill the demands for energy and heat.
3.2.2 Temporal variation profiles
Monthly emissions from power boilers were calculated based on the actual run times of each generator unit.Gas-fired industrial boilers were categorized into a heating season operating type and a year-round operating type with respect to their usage.Natural gas consumption by boilers was calculated based on the capacity and actual run time of each boiler,and residential gas consumption was categorized into heating use and routine cooking use types.The monthly emissions from natural gas combustion were obtained based on the temporal emission distribution.Fig.7 shows the monthly profiles of NOX emission variations in gas-fired combustion,and a comparison to the ambient NO2 concentration in Beijing in 2014 was conducted.
Significant differences were observed in the monthly profiles of NOX emissions from gas-fired combustion.A common feature of these profiles was that NOX emissions in the winter season,which accounted for 59.2% of the annual total,were obviously higher than those in other seasons due to the increased demand for indoor heating.By contrast,the lowest emissions occurred in the summer,when the demand for hot water and steam was lowest.Thus,even the absolute HAPs emissions from natural gas combustion are not as high as those from coal combustion,but the stack height of gas-fired boilers is generally less than 15 meters;thus,the elevated emissions from natural gas combustion combined with unfavorable meteorological conditions in winter undoubtedly contributed to more severe episodes of haze pollution during the winter in Beijing.
NO2 was chosen as a tracer to determine the relationship between the monthly profiles of NOX emissions from gas-fired combustion and the average monthly concentrations of atmospheric NO2 (see Fig.7).Many factors affect the monthly concentrations of air pollutants in the atmosphere,including emissions from industrial sectors,vehicle emissions,meteorological conditions,and so on.Here,we mainly focus on the links between monthly variations in NOX emissions from gas-fired combustion and monthly averaged concentrations of atmospheric NO2.We determined that the monthly NOX emissions from natural gas combustion were not obviously correlated with the monthly ambient concentrations of NO2 based on a nonparametric test.The result demonstrated that the percentage of natural gas combustion in overall emissions is still low,whereas the correlation increased in the heating season,suggesting that the contribution of natural gas combustion to the ambient NO2 concentration increased in this period.This trend should be highlighted and considered in future research and policymaking.

Fig.7 Monthly variations in NOX emissions from gas-fired combustion;monthly average concentrations of atmospheric NO2 in Beijing in 2014
3.3 Uncertainties
Several factors influence the estimation of HAPs emissions from gas-fired combustion in Beijing,including the reliability of activity levels and the adopted EFs of various pollutants.To better understand the uncertainties in our inventory,a Monte Carlo simulation was performed to quantify the potential uncertainties associated with key HAPs emissions from gas-fired facilities.Uncertainties in the activity level and EF data were produced as outputs via simulation equations based on 8,000 rounds of repeated sampling.Detailed information regarding the input parameters and results(expressed as the lower and upper bounds of a 95% confidence interval,CI,around a central estimate)was obtained.The input parameters of specific activity and EFs,with corresponding statistical distributions,were determined based on the authors’ judgments or related published literature(Qiu et al.,2014;Tian et al.,2012;Zhao et al.,2011),which can be seen in supplementary section(Table S1).
Table 2 summarizes the uncertainties in atmospheric emission estimates of HAPs from gas-fired combustion in 2000 to 2014.
Table 2 Uncertainties in the HAPs emissions from gas-fired combustion in Beijing in 2014

As noted above,the emission estimates of several pollutants,such as NOX and CO,for which EFs were determined based on practical monitoring experiments,exhibited relatively small uncertainties.Heavy metals such as Pb,Cd,Hg,Cr,etc.exhibited larger ranges of uncertainty(-96.3%~+93.1%),mainly due to the lack of local EFs determined through adequate field measurements.Therefore,more field tests and surveys are necessary to better understand and quantify the actual emission levels of various HAPs from natural gas combustion.
3.4 Future predictions
According to the Air Pollution Prevention Action Plan issued by the Chinese government(http://www.gov.cn/zwgk/2013-09/12/content_2486773.htm),the maximum concentration of PM2.5 in Beijing should be controlled at approximately 60μg/m3 by 2017 and 35μg/m3 by 2030.To achieve these goals,Beijing will continue to implement its plan for clean energy conversion,which includes a ‘No Coal Area’ strategy.Meanwhile,the consumption of natural gas would continuously increase.All coal-fired industrial boilers would be replaced,and coal-fired power plants would be shut down,assuming a secure supply of natural gas is available.Electricity would be supplied by local gas-fired power plants or long-distance electricity transfer from other provinces.Additionally,residents would use electricity or natural gas for heating and cooking.Promoted by these programs,natural gas consumption in Beijing would reach 28 billion cubic meters in 2030,accounting for 45% of the energy consumption in Beijing under an aggressive energy model.However,because a reliable supply of natural gas cannot be sustained and guaranteed,energy structure adjustments will eventually slow.Natural gas consumption in Beijing is projected to reach 21.7 billion cubic meters based on a conservative energy model and secure energy supply(BMAC,2016).We analyzed and projected future NOX emissions from gas-fired combustion based on different control scenarios and both scenarios of energy consumption growth,as shown in Fig.8.

Fig.8 Scenario analysis of air pollutant emissions from residential coal combustion
In the BAU scenario,we assumed that pollution emissions from in-use or new gas-fired power boilers,industrial boilers,and residential stoves will remain at the current emission levels and that NOX emissions will increase with growing natural gas consumption.NOX emissions from natural gas combustion in 2030 would be 1.9 times and 2.4 times those in 2014 under the conservative and aggressive energy scenarios,respectively.
In the IPC-1 scenario,we estimated that the control of gas-fired boilers would increase in Beijing to meet the air quality improvement and PM2.5 reduction goals.The emission standards of boilers would become more stringent,requiring new gas-fired boilers to install low NOX burners and end-of-pipe controls.Additionally,we assumed that rigorous emission limits would be implemented for in-use boilers at different times,requiring NOX concentrations below 80mg/m3 by 2017 and below 30mg/m3 after 2017.Moreover,relevant economic incentive policies and high-level penalties would be issued,forcing in-use gas-fired boilers to gradually retrofit and reduce their emission levels.In addition,there were no emission limits for residential gas-fired stoves because these limits are difficult to enforce.Consequently,NOX emissions from the residential cooking and heating sector would increase with increasing natural gas consumption.
In the IPC-2 scenario,we estimated that Beijing would implement more aggressive control policies to reduce NOX emissions from gas-fired facilities.Because NOX is one of the important limiting pollutants of air quality,Beijing would adopt more stringent measures for NOX control,and ultra-low NOX gas-fired boilers would be widely developed and installed.In this scenario,NOX emissions would peak in 2017 and then decrease gradually.
3.5 Environmental benefit evaluations of gas-fired and coal-fired boilers
The principle of equivalent substitution of calorific values suggests that 1 ton of coal equals 625m3 of natural gas(He et al.,2017;Niu et al.,2016;Yan et al.,2016).Based on the NOX EFs of coal and natural gas,we compared the emission levels of coal-fired power boilers,industrial boilers,and gas-fired devices in an uncontrolled scenario.Table 3 shows that the NOX emission levels from gas-fired power boilers and industrial boilers decreased to 51.3% and 54.2%,respectively,compared to emissions from coal-fired facilities.As part of the Twelfth Five-Year Plan of China,NOX control technologies were installed in coal-fired power boilers and industrial boilers.Many coal-fired utilities and some coal-fired industrial boilers have adopted low NOX burning and flue purification systems,including SCR,SNCR,and other techniques,to decrease NOX emissions.If emissions from gas-fired combustion are directly released into the atmosphere without control measures,NOX emission levels might surpass those of coal-fired combustion with ultra-low emission control techniques,decreasing the environmental benefits of clean energy conversion.Therefore,in addition to energy substitution,it is quite necessary to lower combustion emissions and even adopts end-pipe emission control technologies at gas-fired facilities.Through combustion control techniques alteration,such as flue gas recirculation(FGR)and low NOX burners and postcombustion technologies(Chen et al.,2017;Ma et al.,2016),NOX emission level of gas-fired facilities could been further reduced,which making the clean energy transformation conducted on combustion facilities to achieve better environmental benefits.
Table 3 Comparison of the NOX emission factors between coal-fired boilers and gas-fired boilers

3.6 Control measures
Natural gas,as a relatively clean energy,plays an important role in air pollution control in Beijing or other regions,energy structure adjustment would benefit for environmental protection.HAPs emission levels from natural gas combustion,such as SO2 and PM levels,have considerably decreased compared to the uncontrolled emissions from coal-fired devices.However,NOX emission levels have only slightly decreased compared to the obvious decreased levels of other HAPs.Currently,the ambient NO2 concentration remains high in Beijing,and the ratio of nitrate to sulfate(/
)in PM2.5 has gradually increased in recent years(Gao et al.,2014,Sun et al.,2016),indicating that local NOX emission levels,as a precursor to PM2.5 and ground-level O3 pollution,are still quite high.Controlling NOX emissions can improve the air quality in Beijing.Unlike coal-fired power boilers that have installed relatively high-efficiency control technologies,such as SCR and SNCR,gas-fired industrial boilers and residential gas-fired stoves generally lack suitable control measures,resulting in higher emissions.
The quality standards of natural gas should be regulated to decrease HAPs emissions from gas-fired combustion.Specifically,the extraction of natural gas should be improved in a manner that reduces the contents of sulfur and heavy metals purities.Based on the available technology,the emission standards for gas-fired devices must be more stringent,forcing the producers of gas-fired devices to implement low NOX combustion technologies,flue gas recirculation,and other control techniques.The owners of gas-fired devices should install flue treatment techniques,such as SCR or SNCR,to reduce emission levels.Creating both economic incentives and penalty mechanisms will encourage emitters to adopt advanced pollution control techniques and reach ultra-low emission levels,while punishing emitters who violate emission limits.Permit and trading systems for pollution source emissions can increase the enthusiasm for emissions reduction and save energy associated with gas-fired combustion.
4 Conclusions
A high-resolution study of emissions from gas-fired combustion in Beijing was performed using a bottom-up method based on available energy data and EFs.In the past year,various control measures have been implemented to reduce the emission of primary air pollutants in Beijing,including shutting down coal-fired plants and replacing coal industrial boilers with natural gas,which has dramatically changed the energy structure.Specifically,the consumption of natural gas has rapidly increased.
NOX emitted from natural gas combustion accounted for approximately 13% of all NOX emissions in Beijing,as well as 23.4% of total NOX emissions in the heating season and 6.2% in the non-heating season.If additional pollution control measures are not taken,the pollutant emissions associated with natural gas combustion will continue to increase,considerably contributing the deterioration of the air quality in and around Beijing.Therefore,HAPs emissions from gas-fired consumption should not be ignored,even as relatively clean energies are substituted for coal in more cities in China.
The best way to control air pollution from gas-fired combustion is to adopt economic incentives that promote de-NOX retrofits,the installation of flue gas purification systems with high removal efficiencies in gas-fired boilers,the strict requirements for gas quality and the use of other combustion controls at current residential gas-fired facilities.Stringent emission standards for gas-fired facilities can force new units to adopt advanced low-NOX combustion,ultra-low NOX combustion,and flue gas purification systems to reduce the final emission levels.
■ACKNOWLEDGMENTS
This work was funded by the National Natural Science Foundation of China(21377012,21177012,and 40975061),the National Science and Technology Support Program of the Ministry of Science and Technology of China(2014BAC 23B02 and 2014BAC06B05),and the Trail Special Program of Research on the Cause and Control Technology of Air Pollution under the National Key Research and Development Plan of China(2016YFC 0201501).
References
[1] BMAC(Beijing Municipal Administration Committee).Plan for natural gas development and construction in the period of 13th Five-Year of Beijing.2016.
[2] BMBS(Beijing Municipal Bureau of Statistics).Beijing Statistical Yearbook.http://www.bjstats.gov.cn/nj/main/2014-tjnj/CH/index.htm.2014.
[3] BMRIEP(Beijing Municipal Research Institute of Environmental Protection).Technical report for emission inventory of primary air pollutants in Beijing,2015.
[4] Chen,H.,Kang,J.N.,Liao,H.,Tang,B.J.,Wei,Y.M.Costs and potentials of energy conservation in China’s coal-fired power industry:A bottom-up approach considering price uncertainties.Energ.Policy 104,23-32.2017.
[5] EEA(European Environment Agency),EMEP/EEA air pollutant emission inventory guidebook-2013.http://www.eea.europa.eu/publications/emep-eea-guidebook-2013.
[6] EPA(Environmental Protection Agency of United States),AP 42 Section 1.4-Natural Gas Combustion.https://www3.epa.gov/ttnchie1/ap42/ch01/final/c01s04.pdf
[7] He,H.,Wang,Y.S.,Ma,Q.X.,Ma,J.Z.,Chu,B.W.,Ji,D.S.,Tang,G.Q.,Liu,C.,Zhang,H.X.,Hao,J.M.Mineral dust and NOX promote the conversion of SO2 to sulfate in heavy pollution days.Sci.Rep.4,4172.2014.
[8] He,G.,Zhang,H.L.,Xu,Y.,Lu,X..China’s clean power transition:Current status and future prospect.Resour.Conserv.Recycl.121,3-10.2017.
[9] Hu,W.W.,Hu,M.,Yuan,B.,Jimenez,J.L.,Tang,Q.,Peng,J.F.,Hu,W.,Shao,M.,Wang,M.,Zeng,L.M.,Wu,Y.S.,Gong,Z.H.,Huang,X.F.,He,L.Y..Insights on organic aerosol aging and the influence of coal combustion at a regional receptor site of central eastern China.Atmos.Chem.Phys.13(19),10095-10112.2013.
[10] Huang,R.J.,Zhang,Y.,Bozzetti,C.,Ho,K.F.,Cao,J.J.,Han,Y.,Daellenbach,K.R.,Slowik,J.G.,Platt,S.M.,Canonaco,F.,Zotter,P.,Wolf,R.,Pieber,S.M.,Bruns,E.A.,Crippa,M.,Ciarelli,G.,Piazzalunga,A.,Schwikowski,M.,Abbaszade,G.,Schnelle-Kreis,J.,Zimmermann,R.,An,Z.,Szidat,S.,Baltensperger,U.,Haddad,I.E.,Prevot,A.S.H..High secondary aerosol contribution to particulate pollution during haze events in China.Nature 514(7521),218-222.2014.
[11] Gao,J.J.,Tian,H.Z.,Cheng,K.,Lu,L.,Wang,Y.X.,Wu,Y.,Zhu,C.Y.,Liu,K.Y.,Zhou,J.R.,Liu,X.G.,Chen,J.,Hao,J.M..Seasonal and spatial variation of trace elements in multi-size airborne particulate matters of Beijing,China:Mass concentration,enrichment characteristics,source apportionment,chemical speciation and bioavailability.Atmos.Environ.99,257-265.2014.
[12] Lin,A.J.,Pu,Y.,Qi,W.K.,Li,X.L.,Li,X.J.,Wang,C.F.,Yang,X.J.,China’s partial emission control.Science 351(6274),674-675.2016.
[13] Liu,J.H.,Chen,S.L.,Wang,H.,Campana,P.E.,Yan,J.Y..Evolution of China’s Urban Energy Consumption Structure—A Case Study in Beijing.Energ.Proc.88,88-93.2016.
[14] Liu,J.,Mauzerall,D.L.,Chen,Q.,Zhang,Q.,Song,Y.,Peng,W.,Klimont,Z.,Qiu,X.H.,Zhang,S.Q.,Hu,M.,Lin,W.L.,Smith,K.R.,Zhu,T..Air pollutant emissions from Chinese households:A major and underappreciated ambient pollution source.Proc.Natl.Acad.Sci.USA 113(28),7756-7761.2016.
[15] Makonin,S.,Ellert,B.,Baji'c,I.V.,Popowich,F..Electricity,water,and natural gas consumption of a residential house in Canada from 2012 to 2014.Sci.Data 3,160037.2016.
[16] Ma,Z.Z.,Deng,J.G.,Li,Z.,Li,Q.,Zhao,P.,Wang,L.G.,Sun,Y.Z.,Zheng,H.X.,Pan,L.,Zhao,S.,Jiang,J.K.,Wang,S.X.,Duan,L..Characteristics of NOX emission from Chinese coal-fired power plants equipped with new technologies.Atmos.Environ.131,164-170.2016.
[17] McJeon,H.,Edmonds,J.,Bauer,N.,Clarke,L.,Fisher,B.,Flannery,B.P.,Hilaire,J.,Krey,V.,Marangoni,G.,Mi,R.,Riahi,K.,Rogner,H.,Tavoni,M..Limited impact on decadal-scale climate change from increased use of natural gas.Nature 514(7523),482-485.2014.
[18] Niu,S.W.,Liu,Y.Y.,Ding,Y.X.,Qu,W..China’s energy systems transformation and emissions peak.Renew.Sustain.Energy Rev.58,782-795.2016.
[19] Pei,B.,Wang,X.L.,Zhang,Y.H.,Hu,M.,Sun,Y.J.,Deng,J.,Dong,L.,Fu,Q.Y.,Yan,N.Q..Emissions and source profiles of PM2.5 for coal-fired boilers in the Shanghai megacity,China.Atmos.Pollut.Res.7(4),577-584.2016.
[20] Qiu,P.P.,Tian,H.Z.,Zhu,C.Y.,Liu,K.Y.,Gao,J.J.,Zhou,J.R..An elaborate high resolution emission inventory of primary air pollutants for the Central Plain Urban Agglomeration of China.Atmos.Environ.86,93-101.2014.
[21] SEPA(State Environmental Protection Administration).Manual of industrial pollution source emission coefficient in the first national pollution census.China Environmental Science Press.2008.
[22] Sun,Y.L.,Wang,Z.F.,Wild,O.,Xu,W.Q.,Chen,C.,Fu,P.Q.,Du,W.,Zhou,L.B.,Zhang,Q.,Han,T.T.,Wang,Q.Q.,Pan,X.L.,Zheng,H.T.,Li,J.,Guo,X.F.,Liu,J.G.,Worsnop,D.R.,“APEC Blue”:Secondary Aerosol Reductions from Emission Controls in Beijing.Sci.Rep.6,20668.2016.
[23] Tian,H.Z.,Gao,J.J.,Lu,L.,Zhao,D.,Cheng,K.,Qiu,P.P..Temporal trends and spatial variation characteristics of hazardous air pollutant emission inventory from municipal solid waste incineration in China.Environ.Sci.Tech.46(18),10364-10371.2012.
[24] Tian,H.Z.,Wang,Y.,Xue,Z.G.,Cheng,K.,Qu,Y.P.,Chai,F.H.,Hao,J.M..Trend and characteristics of atmospheric emissions of Hg,As,and Se from coal combustion in China,1980-2007.Atmos.Chem.Phys.10(23),11905-11919.2010.
[25] Wang,S.X.,Zhang,L.,Li,G.H.,Wu,Y.,Hao,J.M.,Pirrone,N.,Sprovieri,F.,Ancora,M.P..Mercury emission and speciation of coal-fired power plants in China.Atmos.Chem.Phys.10(3),1183-1192.2010.
[26] Xue,Y.F.,Tian,H.Z.,Yan,J.,Zhou,Z.,Wang,J.L.,Nie,L.,Pan,T.,Zhou,J.R.,Hua,S.B.,Wang,Y.,Wu,X.Q..Temporal trends and spatial variation characteristics of primary air pollutants emissions from coal-fired industrial boilers in Beijing,China.Environ.Pollut.213,717-726.2016.
[27] Xue,Y.F.,Nie,T.,Zhou,Z.,Yan,J.,Nie,L.,Emission of NOX from gas-fired industrial boilers in Beijing and their Impact on air quality.Huan jing ke xue yu ji shu 37 118-122(in chinese).
[28] Yan,B.F.,Xue,S.,Li,Y.F.,Duan,J.H.,Zeng,M..Gas-fired combined cooling,heating and power(CCHP)in Beijing:A techno-economic analysis.Renew.Sustain.Energy Rev.63,118-131.2016.
[29] Zhang,J.,Li,L.,Yu,L.,Tang,L..Comprehensive evaluation of energy intensity change for 1997-2012 based on input-output analysis:Evidence from Beijing.Proc.Comp.Sci.91 1057-1063.2016.
[30] Zhao,Y.,Nielsen,C.P.,Lei,Y.,McElroy,M.B.,Hao,J.M..Quantifying the uncertainties of a bottom-up emission inventory of anthropogenic atmospheric pollutants in China.Atmos.Chem.Phys.11(5),2295-2308.2011.