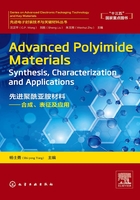
2.4 Structure and Properties of Polyimide Fibers
2.4.1 Aggregation Structure of Polyimide Fibers
PI fibers possess several distinguished characteristics, such as high modulus, high strength, excellent thermal dimensional stability and chemical resistance, which are not only the result of the rigid-rod polymer backbones but also of the highly oriented polymer chains and ordered lateral packing. PI materials are always regarded as the semi-crystalline polymers. However, crystallinity and orientation degree of PI fibers would increase clearly, and typical crystalline regions would form in the fibers after the thermal stretching process, which are the main factors for preparing high-strength and high-modulus PI fibers. As reported by Wakita et al. [53], common PI materials are amorphous, which means that most PI cannot form 3D crystalline structures. Saraf [54] thought PIs tend to form “liquid-crystalline-like ordered regions” (LC-like). The packing of PI chains was relatively disordered in a liquid-crystalline-like ordered structure due to variation in conformation around the chain axis and the distribution of interchain distances. In the amorphous region, molecular chains are in a random arrangement, and charge transfer complexation occurs between electron-donating diamine moieties and electron-accepting dianhydride moieties, which easily form “mixed layer packing”. Wu and coworkers [55] has proposed a hypothetical structure model of the PI fibers as pictured in Fig. 2.19. The co-PI fibers are composed of fibrils and exhibit skin-core structure due to the different solidification rates between the external and inner structure. The packing compactness of the fibrils near the surface is higher than that in the core, resulting in different abilities of deformations between the skin to the core in the fibers at high DRs. For the ordered structure region, the molecules tend to align in a distinct series of layers, while there are only liquid-like short-range order within their layers, which is named a “smectic a liquid crystal-like” ordered layer structure. The ordered chain packing along the c-axis and the short-range ordered lateral packing in the ab-plane are formed.

FIGURE 2.19 The sketch of a hypothetical structure model of the copolyimide fibers.
Crystalline structures and lattice parameters of PI fibers have been investigated in detail [44]. A novel PI fiber has been spun based on BPDA and DMB via the dry-jet wet-spinning. The fibers were stretched and annealed at elevated temperatures above 400 °C to achieve excellent mechanical properties. As for seven-times drawn fibers, the BPDA-DMB molecular packs into a triclinic unit cell with dimensions of a=2.10(2) nm, b=1.523(8) nm, c=4.12(7) nm, α=61.2°, β=50.7°, γ=78.9°, and the number of chain repeating units per unit cell (Z) is 16. After annealing at elevated temperatures, the unit cell of fibers changed a little, that is a=2.048(6) nm, b=1.529(5) nm, c=4.00(2) nm, α=62.1°, β=52.2°, γ=79.6°. After heat-drawing and annealing treatment, both the crystallinity and crystal orientation increase. Obata et al. [56] prepared a wholly aromatic PI fiber with a rigid-rod chain based on PMDA and benzidine (BZ) and analyzed the molecular conformation and crystal structure of fibers using WAXD measurement and computer simulation. The unit cell parameters were a=0.857 nm, b= 0.551 nm, c=1.678 nm, and the crystalline structure belonged to an orthorhombic crystal system.
Evolution of the aggregation structure of co-PI fibers containing flexible and rigid diamines in the heat-drawing process has been investigated in recent years. Zhang’s group prepared a series of co-PI fibers by introducing a new rigid diamine 2,6-(4,4′-diaminodiphenyl) benzo[1,2-d:5,4-d′] bisoxazole (PBOA) into the relative flexible homo-PI backbones of BPDA and ODA. In Fig. 2.10, all of the as-spun fibers exhibited obscure equatorial arcs, named “amorphous halos,” indicating relatively low ordering degrees of the lateral stack of the polymer chains in the as-spun fibers. However, by thermal treatment, significant changes appear in the fibers’ diffraction patterns. All drawn PBOI fibers had similar lattices with the BPDA-ODA oriented PI thin films showing orthogonal geometry. Several [001] diffractions appear on the meridian (drawing direction) and obscure [hkl] diffractions can be observed in the quadrants, reflecting at least with the lattice parameters of α=β=90°. Furthermore, the appearance of the [hk0] planes on the equator can be explained by assuming γ =90°. Accordingly, it can be assigned that the crystal structure of these PBOI fibers is orthorhombic symmetry. The distinct diffraction arcs of (001) lattice planes along the meridian represent the rigid-rod polymer chains having a very high degree of preferred orientation aligned with the fiber axis. Meanwhile, equatorial reflections become much sharper and several clear diffraction points can be observed indicating the formation of a well-defined intermolecular lateral packing order, which means that the regularity of the molecular stacking has been substantially improved and crystalline regions have formed in the thermal drawn PBOI fibers.
Meanwhile, by means of small-angle X-ray scattering (SAXS), Yin et al. [57] intensively investigated the morphology evolution of the PI fibers. The micromorphology of PI fibers changes dramatically by introducing a relatively rigid diamine, PBOA, into the BPDA-ODA backbones. As shown in Fig. 2.20, the intense and elongated streaks near the beam stop along the meridian direction reflecting the oriented needle-like structures of microvoids which aligned parallel to the fiber axis. After the thermal stretching, for all resulting fibers, the scattering pattern exhibits a sharp and elongated equatorial streak superimposed with an obvious meridional intensity distribution, suggesting the formation of periodic lamellar structures in the drawn PBOI fibers.

FIGURE 2.20 2D WAXD patterns of BPDA/ODA/PBOA copolyimide fibers (molar ratio: (A) ODA/PBOA=9/1; (B) 8/2; (C) 7/3; (D) 6/4; (E) 5/5).
Additionally, this meridional intensity becomes stronger with increasing PBOA concentrations. 1D SAXS scattering profiles of PI fibers along the stretching direction are shown in Fig. 2.21B; a scattering maximum is consistently seen in all fibers, which can be attributed to the long period repeat from a crystalline and amorphous two-phase stacking morphology. The scattering maximum gradually moves to a lower q value with increasing PBOA content. The long period L obtained from the correlation function ranges from 19.3 Å to 22.0 Å, and increasing PBOA content will result in higher long periods. In addition, it is seen that the calculated crystal thickness 1C and amorphous layer thickness lA slightly increase with increasing PBOA content, which are in the range of 8.2 Å-9.3 Å and 11.1 Å-12.8 Å, respectively, based on the correlation function as shown in Fig. 2.21C.

FIGURE 2.21 (A) 2D SAXS patterns of the BPDA/ODA/PBOA copolyimide fibers; (B) 1D SAXS scattering intensity distribution of PBOI fibers along stretching direction; (C) corresponding correction function diagrams calculated from Fig. 2.21B.
2.4.2 Chemical Structure-Property Relationship
As is known that subtle variations in the structures of the dianhydride and diamine components have a tremendous effect on the properties of the final PI fibers. Different chemical composition directly leads to various performances of the PI fibers. Herein, we focused on the relationship between chemical structure and properties.
2.4.2.1 Typical Homo-Polyimide Fibers
Among all the preparation systems, the PMDA/ODA system is considered as the simplest one due to the high reactivity of the two monomers when reacted with other dianhydrides/diamines. The PMDA/ODA polymer backbone consists of rigid PMDA units and flexible ODA units, and thus leading to the contradiction in the performance of the resultant PI fibers. The rigid polymer chains gave rise to the excellent thermal-oxidative stability with 10% weight loss temperature (Td10) over 540 °C in air atmosphere and glass transition temperature of 410 °C. On the other hand, the flexible ODA units contorted the chain symmetry and thus made it harder for the chain to pack into the crystal lattice, resulting in a poor degree of lateral molecular arrangement. The fibers derived from PMDA/ODA system exhibited the tensile strength, initial modulus and elongation up to 0.4 GPa, 5.2 GPa, and 11.1%, respectively. The PI fibers derived from BPDA and p-PDA are preferred due to the stiff and linear chain structure, strong intermolecular association, and high molecular orientation. The fibers prepared in BPDA/PDA system possessed the tensile strength, initial modulus, and elongation of 1.07 GPa, 50.39 GPa, and 1.12%, respectively, with the Tg around 340 °C [35]. However, the stiffness polymer chains resulted in the poor processability of the fibers simultaneously. The BPDA/ODA PI fibers exhibited the tensile strength and initial modulus of 0.8 GPa and 30 GPa with the drawing ratio of 5.5. The fibrils and microvoids could be identified from the fractured cross-sectional morphologies, which had affected the mechanical properties of the PI fibers. The fibers also exhibited excellent thermal stabilities with the Td5 around 400 °C in air atmosphere, a negative value of linear coefficient of thermal expansion below 400 °C, and Tg ranged from 276 °C to 297 °C with the frequency increased from 0.1 Hz to 100 Hz.
2.4.2.2 Copolyimide Fibers with Hydrogen Bonding Interactions
The heterocyclic diamine, AAQ or BIA, is a versatile monomer with an asymmetry structure feature, which has strong inter-or intramolecular hydrogen bonding formation capability that strengthens the mechanical properties of resulting fibers. The hydrogen bonding interactions are usually investigated by deconvoluting the Fourier transform infrared (FTIR) spectra in a wave number range of 1700cm-1-1750 cm−1. For instance, the percentage of the hydrogen bonding associated imides in the BPDA/PDA/AAQ co-PI fibers increases with increasing AAQ content and the AAQ moiety has a positive effect on preventing the side reactions during the thermal cycloimidization process [39]. The hydrogen bonding effectively enhanced the mechanical properties of the co-PI fibers, reaching the optimum tensile strength of 2.8 GPa and modulus of 115 GPa, at the AAQ/PDA molar ratio of 5/5. The 5% weight loss temperatures (Td5) of the BPDA/PDA/AAQ fibers under nitrogen were in the range of 599 °C-604 °C, implying that hydrogen bonding interactions also play a positive role in improving the thermal stability of the PI fibers. Moreover, the fibers exhibited gradually enhanced glass transition temperatures (Tg) from 340 °C to 400 °C with increasing AAQ content. The BPDA/PDA/AAQ co-PI fibers possessed a highly ordered structure along the fiber direction with the optimum orientation degree of 0.86. Moreover, the ordered chain repeat length was slightly decreased with the incorporation of quinazolinone moiety in the co-PI fibers, ascribing to the relatively flexible structure of the AAQ unit.
Dong et al. [58] investigated the effects of TFMB/BIA molar ratio on the structures and properties of BTDA/TFMB/BIA fibers. The heterocyclic diamine BIA provided a strong intermolecular hydrogen bonding interaction that strengthened the mechanical properties of the PI fibers, while the diamine TFMB possessed a rigid nonplanar structure with two bulky trifluoromethyl groups, resulting in great benefits for improving solubility of the PIs. Consequently, the final PI fibers exhibited the tensile strength of 2.25 GPa and modulus of 102 GPa with a drawing ratio of 3.0 when the molar ratio of TFMA/BIA was 50/50. The fibers exhibited excellent thermal stabilities in the range of 531 °C-550 °C in nitrogen and 528 °C-544 °C in air for a 5% weight loss. Meanwhile, the improved thermal stability with more BIA contents is related to the increased intermolecular interactions and crystal structures. And the corresponding Tgs of the fibers displayed a clear increasing trend with increasing BIA content, reaching a value of 360 °C. Similarly, the PI fibers derived from BPDA, 4-amino-N- (4-aminophenyl) benzamide (DABA) and BIA possessed the tensile strength and initial modulus up to 1.96 GPa and 108.3GPa, respectively, attributed to the well-defined ordered and dense structure. The chemical structure and molecular packing significantly affected the thermal stability of fibers, resulting in gradual increased Tgs from 350 °C to 380 °C with increasing DABA content [59].
It is pointed out that those hydrogen bonding interactions would act as physical cross-linking points and lead to the benzimidazole/imide “mixed layer” packing in which the imide ring of one chain and the (amine) phenyl ring of another chain adopt a coplanar “sandwich” conformation. The crystallinity of the fibers enhanced from 1.8% to 24.5% when the annealing temperatures increased from 390 °C to 400 °C, which was manifested as a sudden crystallization process. The increased crystallinity of the fibers led to the improvement in mechanical properties simultaneously, and the tensile strength increased from 1.31 GPa to 1.68 GPa in the same temperature range.
2.4.2.3 Copolyimide Fibers Containing Ether Units
The incorporation of hydrogen bonding interactions greatly enhanced the mechanical properties of PI fibers. Nevertheless, with the increased rigid units, it is difficult for the polymer chains to rearrange themselves to reduce the size of microvoids and for fiber spinning. On the contrary, the introduction of flexible units into the rigid polymer backbone can provide opportunities to reduce the size of microvoids because of the increased flexibility of polymer chains. For instance, the fibers containing ODPA moieties exhibited the tensile strength and initial modulus in the range of 1.07GPa-1.58 GPa and 50.39 GPa-67.75 GPa, respectively. The optimum mechanical properties of the PI fibers containing ODA contents were obtained with the tensile strength of 2.53 GPa at a PDA/ODA molar ratio of 5/5. The BPDA/PDA/ODA fibers exhibited thermal stabilities of up to 563 °C in nitrogen and 536 °C in air for a 5% weight loss and Tgs above 270 °C The average radius, length, misorientation, and internal surface roughness of microvoids were found to decrease with increased ODPA or ODA contents, suggesting the gradually formed homogenous structures in the fibers and accounting for the improved mechanical properties. However, the values of Td5 both in nitrogen and air reduced with increasing ODPA or ODA content, which is attributed to the increased C-O bonds in the polymer chains because the C-O bonds are much more easily broken than C-C bonds. And the values of Tg and tan delta exhibited a clear decreased and increased tendency, respectively, due to the increased flexibility of the polymer chain.
The effect of the BIA and ODA moieties on the properties of the PI fibers was systematically investigated. The results showed that both the introduction of BIA and ODA could improve the mechanical properties of PI fibers, but the improvement induced by BIA moieties was larger than that of ODA moieties. For instance, the fibers with the PDA/BIA/ODA molar ratio of 6/1/3 possessed the tensile strength and initial modulus of 2.19 GPa and 60.85 GPa, while the value increased to 2.72 GPa and 94.33 GPa when the molar ratio of p-PDA/BIA/ODA was 6/3/1, respectively. Due to the different mechanisms in improving the mechanical properties of PI fibers, the fibers with more ODA moieties exhibited the more disrupted lateral molecular packing in the fibers and reduced thermal-oxidative stabilities. However, the case was on the contrary for the BIA moiety. The fibers exhibited excellent thermal and thermal-oxidative stability, with a Td5 of 578 °C in nitrogen and 572 °C in air.
2.4.2.4 Copolyimide Fibers Containing Benzoxazole Units
The heterocyclic benzoxadazole moiety has an analogous chemical structure to benzimidazole and also shows unique properties. Inspired by the fact that Zylon fiber (PBO) containing benzoxazole units possesses very high tensile strength of 5.8 GPa, modulus of 270 GPa, excellent thermal stability as well as good environmental resistance, the introduction of benzoxazole units is expected to enhance the mechanical and thermal properties, as well as decrease the water absorption of the PI fibers. Huang et al. introduced 2-(4-aminophenyl)-5-aminobenzoxazole (BOA) into the BPDA/p- PDA polymer backbone [60]. The mobility of the polymer chains was improved with the incorporated third monomer and favored large drawing during the thermal treatment process and reduced the influence of the voids. Therefore, the mechanical properties were subtly improved, reaching the tensile strength of 1.0 GPa and modulus of 92 GPa. However, further addition of the third monomer reduced the stacking density of the polymer chains oppositely, which was not conducive to the improvement in the mechanical properties of PI fibers.
Yin et al. [61] investigated the effects of different molar ratios of BIA/BOA on the performances of the BPDA/BIA/BOA PI fibers. The optimal mechanical properties of co-PI fibers were obtained when the molar ratio of BIA/BOA was 7/3, with a tensile strength and modulus of 1.74 GPa and 74.4 GPa, respectively. SAXS results illustrated that addition of BOA accounts for the disappearance of the periodic lamellar structure for homo-BPDA/BIA PI fibers. Co-PI fibers with the molar ratio of BIA/BOA=9/1 and 7/3 have the smallest radius of the microvoids, suggesting the most homogenous structure. The prepared co-PI fibers showed thermal stability with Tgs ranging from 367 °C to 302 °C, and Td5 in N2 is 540 °C-530 °C. The corresponding Tgs of the fibers displayed a clear decreasing trend with increasing BOA content. Additionally, the hydrophobic behavior of the composite fibers is greatly enhanced by incorporating BOA content so that the fibers can be employed in the hot and humid environment. Sun et al. investigated the effects of BIA/BOA molar ratios on the properties of BPDA/PDA/BIA/BOA co-PI fibers [62]. Due to the presence of hydrogen bonding interaction and high orientation in the fibers, the mechanical properties of the BPDA/PDA/ BIA/BOA fibers were improved when both BOA and BIA were incorporated into the polymer chains. The optimum tensile strength was 2.26 GPa with the BIA/BOA molar ratio of 3/1, whereas the optimum initial modulus was 145.0 GPa with the BIA/BOA molar ratio of 1/3. Moreover, the co-PI fibers exhibited excellent thermal properties with 5% weight loss temperature ranging from 563 °C to 570 °C in nitrogen and glass transition temperature ranging from 308 °C to 321 °C.
2.4.2.5 Copolyimide Fibers Containing Fluorinated Groups
The reduced dielectric permittivity of PI fibers is of significance when occupied in microelectronic industries. There is thus no doubt that the introduction of fluorine groups is one of the most efficient approaches to reducing the dielectric permittivity of the PI fibers due to the low molar polarization ratio of the F atom. The 4,4’-(hexafluoroisopropylidene)diphthalic anhydride (6FDA)/2,2-bis(4-(4-aminoph- enoxy(-phenyl)-hexa-fluoropropane (4BDAF) fibers had a maximum tensile strength and modulus of 200 MPa and 6 GPa, respectively. A dielectric constant of 2.5 was measured over a frequency range between 1.0 MHz and 1.8 Hz. Also, the BPDA/PDA/TFMB fibers prepared by Huang et al. [41] showed enhanced mechanical properties with increased TFMB contents. It is stated that the mobility of polymer chains could be improved, thereby a larger drawing ratio could be obtained in the heat-treatment stage. Chang et al. [63] have investigated the effects of 6FDA on the properties of the BPDA/6FDA/PDA/ODA/BIA co-PI fibers. The increased 6FDA moieties in the system resulted in unexpected great changes in the properties of the resultant PI fibers. Regarding mechanical performances of the PI fibers, the tensile strength and initial modulus of the fibers decreased from 2.56 GPa to 0.13 GPa and 91.55 GPa to 2.99 GPa, respectively. The PI fibers decomposed at lower temperature with the increased 6FDA contents, which was attributed to the decreased stacking density and increased free volume of the polymer chains induced by the introduction of ‒CF3 pendant group. The dielectric permittivity was found to decrease from 3.46 to 2.78 in the frequency of 10 MHz with the increased 6FDA contents, associated with the increased free volume of the polymer chains and macrovoids structures in the fibers.
2.4.3 Properties of Polyimide Fibers
2.4.3.1 Mechanical Properties
Tensile strength and modulus of PI fibers mainly depend on the chemical structure, molecular weight, orientation degree, and crystallinity, as well as the dispersion of defects of the fibers. At present, the tensile strength and modulus of heat-resistant PI fibers are in the range of 0.5 GPa-1.0 GPa and 10 GPa-40 GPa, respectively. However, for the high-strength and high-modulus PI fibers, the tensile strength is over 2.5 GPa and the modulus is as high as 90 GPa. Fig. 2.22 compares the tensile properties between PI fibers containing pyridine units and several commercial polymeric fibers [64]. Apparently, PI fibers show higher specific strength and specific modulus than the well-known Kevlar 29, Twaron fibers and so on, and the specific strength is even higher than Kevlar 49, which indicates a wide application prospect in the area of composite materials.
FIGURE 2.22 Comparison of specific strength and modulus of PI fibers containing pyridine units with other polymeric fibers.
2.4.3.2 Thermal Stability
For the wholly aromatic PI fibers, the initial decomposition temperatures are generally over 500 °C, and a specific PI fiber synthesized from BPDA/p-PDA shows an initial decomposition temperature of over 600 °C, which is one of polymers with the best thermal stability properties.
2.4.3.3 Chemical Resistance
PI fibers show excellent chemical resistance in an acidic environment. Fig. 2.23 shows the change of tensile strengths of three different PI fibers (PI-1, PI-2, and commercial P84 fiber) and the Poly(phenylenesulfide) (PPS) fiber after treatment in a 10%(vol) HCl aqueous solution at different times. It is obvious that the strength retention of PI-1 and PI-2 fibers are as high as 30%-40%, which is much better than the P84 fiber of Evonik. Unfortunately, all PI fibers show poor resistance in an alkaline environment, in which the polymer chains will degrade rapidly. However, this characteristic also brings some benefits for PI: for example, the diamine and dianhydride monomers of PI fibers can be recycled in a base solution.

FIGURE 2.23 Tensile strength of PI fibers, P84 fiber and PPS fiber as a function treating time in a 10 %(vol) HCl aqueous solution (PI-1 was the thermal treated fiber provided by Jiangsu Aoshen Hi-tech Materials Co., Ltd, and PI-2 fiber was the as-spun fiber).
2.4.3.4 Flame-Retardant Properties
PI fibers have been regarded as one of the most excellent flame-retardant materials and exhibit outstanding self-extinguishing performance, which can satisfy the flame-retardant requirements in most areas. Meanwhile, the fire-resistant properties of PI fibers can be affected by the chemical structures; for example, the limiting oxygen index (LOI) for PMDA/ODA PI is around 37%, while, for BPDA-p-PDA, the LOI reaches 66%, satisfying the application of PI fibers in some special environments.
2.4.3.5 Other Properties
Most PI fibers possess excellent radiation resistance, and the strength retention can reach 90% after being irradiated under a 1×108 Gy fast electron irradiation. Meanwhile, PI fibers have superior dielectric properties and the dielectric constant of the PMDA-ODA PI fiber is around 3.4. By introducing fluorine units or bulky pendant groups, the dielectric constant can be as low as 2.8-3.0, and dielectric loss reaches around 10−3, making PI fibers ideal reinforcements for wave-transparent composites.